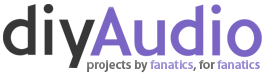
|
|
"Shockley's relation states that the short-circuit {emitter} input resistance of an ideal transistor varies inversely with the emitter current and is 26 ohms when the emitter current is 1mA at room temperature." {Analysis and Design of Transistor Circuits, Cowles, 1966, van Nostrand}
Yeah, so?
Well, for complicated reasons, a good Silicon transistor is more like 28 or 30 ohms, but is otherwise darn close to Shockley's "ideal transistor" over a very wide range of current.
The dynamic resistance of the emitter at various currents is:
30,000 ohms at 1ľAmp
3,000 ohms at 10ľAmp
300 ohms at 100ľAmp
30 ohms at 1 milliAmp
3 ohms at 10 milliAmp
0.3 ohms at 100 milliAmp
This is true for ANY Silicon bipolar transistor.
Does not matter what size it is, big or small, except that small devices may have an Ohm of parasitic resistance and large devices may have 1ľAmp leakage. You can try to find the actual value of parasitics and leakage, but in practice you just stay well inside the maker's limits and you will probably be fine. A 100mA device will follow Shockley's relation up to 25 or 50mA. (This does get a little "wrong" when you get to Power devices: a "15 Amp" device working at 10 Amps will have 0.003 ohms Shockley resistance and maybe 0.1 ohms parasitic resistance.)
Oh, the Transconductance (Gm) is the inverse of the emitter resistance. 30 ohms at 1 mA is 0.03 Mho or 30,000 ľMhos. Personally, I'm a ratiometric kind of guy: I like to work with resistance ratios instead of multiplying by Transconductance. But the answer comes out the same either way.
What else do when know when we grok Shockley?
The maximum voltage gain of a resistance-loaded transistor amp is about 30*Vcc, where Vcc is the supply voltage. Why? If we have a 10 volt supply, set current at 1mA, the max Rl is not-quite 10K (any more and we can't get 1mA). The voltage gain is Rl/Re. Rl is 10K, Re is 30, the voltage gain is 10,000/30= 300. If we try 10ľAmp instead, the max Rl is 1Meg, the Re is 3,000, 1,000,000/3,000= 300, same as at 1mA. So if we have a fixed supply and want to resistor-load, there is no point in asking for more than 30*Vcc in one stage. Can't happen. And in practice, we usually want some signal swing: if we set the bias point to 0.5*Vcc (max voltage swing) the max gain drops to 15*Vcc. If you need more gain, you either need another stage, or a non-resistor (active) load (and probably a buffer too). (Going to very high Vcc falls-short for a different reason. The Early Effect, Reverse Transfer Ratio, what triode-heads call Amplification Factor, for most transistors is 200 to 1,000. We can cascode that away, though gains of 1,000+ in one place tend to be tricky in many ways.)
GM ("gain") varies with current. In a resistor loaded stage, current varies with output signal voltage. Therefore gain varies with signal voltage. If we hope to swing from Vcc/2 up almost to Vcc and down almost to zero, Gm will fall to zero near Vcc and rise to double near zero volt out. But for distortionless audio, gain must not vary with signal voltage. Gain falling to zero means one side will be flattened, and gain rising to double will make the other side bump-up grotesquely. A resistance loaded voltage amp's THD quickly rises to 10% and 25%, considerably short of a full swing.
Ah, we can "fix" that with added resistance in the emitter leg. With 1Ma current, Re about 30 ohms, adding 1O fixed resistance will make no real change, 30 ohms fixed R will cut the distortion (and gain) about in half, and 300 ohms will cut distortion (and gain) to 1/10th of a naked transistor. So you need an estimate of emitter dynamic impedance to estimate the size of emitter resistor you will need to meet a given linearity spec, and to estimate gain-error at high (for the supply voltage) gains. You may not be estimating Gm (or Hib) directly, but if your transistors always do what you want when you stick resistors under their emitters, you must have some sense of the magnitude of Gm or Hib.
What is the input impedance of a grounded-emitter transistor amplifier? Just Beta times Hie (in parallel with any bias and coupling networks). At 1mA, Hie=30 ohms, Beta say 100, the input impedance is 30*100= 3K ohms. You can raise this with higher Beta, though that (used to) costs more and Beta has limits. You can use an emitter resistor, also reducing gain and distortion. Or you can run the transistor at a lower current: 10ľA with same Beta gives input impedance of 300,000 ohms. Since the voltage gain can stay the same, this is what most input stages do, though they will need another stage to buffer any load that wants more than 10ľA drive current.
If Hie runs 30 ohms at 1mA, how-come the Base-Emitter voltage drop isn't 0.030V, but more like 0.6V? Well, work backward. At 0.1mA it is 300 ohms, at 0.01mA it is 3,000 ohms. If you follow this logic all the way to zero current, you get infinite Vbe. Huh? You need to know that any junction has a small internal current, and Shockley's relation for a diode only holds down to that current. That current (intrinsic saturation current, I(sat)) is rarely specified, but if you know it the actual Vbe can be closely estimated from Shockley and the I(sat). I(sat) does depend on area, which is why two devices of different areas have different Vbe at the same current.
And from that, you get an intuitive (not rigorous) understanding of the very useful fact that the Vbe of a Silicon junction rises 60mV for each decade of current (20mV for each doubling of current). If you want a current mirror to reflect double the current, you need to inject 20mV into one side. That's also handy when biasing the standard push-pull emitter follower beloved of op-amp and speaker-amp designers.
A full derivation of Shockley also involves Temperature. The 6mV/°C slope of diode or transistor junction drop falls right out of Shockley's relation.
Ebbers-Moll contains Shockley but covers the very-low range (below I(sat)) and the three whole quadrants where bias voltages are "backward" to how we use transistors in "linear" circuits. It may not be necessary in common-sense design of linear circuits, but the Ebbers-Moll equations are starkly simple considering how well they predict behavior of actual transistors over all the extremes.
There are no such simple universal equations for vacuum tubes or FETs. Over a useful range, these can be modeled with simple exponentials (Square Law, Three-Halves Law). But FETs deviate from Square Law at "useful" currents, and no commercial vacuum tube really obeys the Three-Halves Law (this seems to have been an un-said secret at the time vacuum tube development stopped, and I have not seen a clear explanation of real commercial VT curves). And the absolute value of the parameters is totally up to size and construction, they do not have a universal relation like the BJT does.