Well certainly the CLD approach can work, and work well... but I see it as difficult to execute in a one-off cabinet.
The CLD approach is difficult to simulate well. It has to be empirically verified. Only with a regimen of prototyping, testing, and redesign is it possible to fine tune the damping of a system (speaker cabinet or other structure). If you are able and willing to measure the cabinet with accelerometers, open it up to add/remove damping, add/remove bracing, re-assemble, re-test... at least several times... well, yes, you can very likely achieve a non-resonant cabinet. The speaker manufacturers who successfully build CLD cabinets use this approach.
A high-stiffness approach, on the other hand, is a method which is easier to achieve first-time quality. For a given cabinet shape and dimension, it is a pretty straightforward process to design a bracing scheme that will result in a non-resonant cabinet. The constructor of a one-off cabinet can have high confidence that he won't have to repeatedly modify the cabinet to get it right.
The CLD approach is difficult to simulate well. It has to be empirically verified. Only with a regimen of prototyping, testing, and redesign is it possible to fine tune the damping of a system (speaker cabinet or other structure). If you are able and willing to measure the cabinet with accelerometers, open it up to add/remove damping, add/remove bracing, re-assemble, re-test... at least several times... well, yes, you can very likely achieve a non-resonant cabinet. The speaker manufacturers who successfully build CLD cabinets use this approach.
A high-stiffness approach, on the other hand, is a method which is easier to achieve first-time quality. For a given cabinet shape and dimension, it is a pretty straightforward process to design a bracing scheme that will result in a non-resonant cabinet. The constructor of a one-off cabinet can have high confidence that he won't have to repeatedly modify the cabinet to get it right.
More thoughts...
I suspect that there is a resistance to accept the high-stiffness approach because it seems counter-intuitive.... The thinking goes something like this: Energy pumped into the cabinet walls by the driver is either radiated, or it is absorbed. Adding stiffness does not help absorb the energy... only by damping the vibrations and converting them into heat do we prevent re-radiation of that structural vibration as unwanted sound.
If speaker was required to produce continuous sine wave or noise signals, this argument would be (basically) true. However, that is not what speakers do.
The reason the high stiffness approach works is two-fold: (1) the vibration forcing function is music or movie soundtrack... which contains lots of transients. The frequency range of music that contains continuous high energy is approximately 50 Hz to 500 Hz. Above that range, the energy comes in short bursts. (2) second reason high stiffness works... At higher frequencies, all structures have increasing self damping. Plywood, MDF, and most other common materials have a lot more damping at 1 kHz than they do at 100 Hz. Same is true for glues and adhesives.
Planet10 has made this argument quite a few times in quite a few threads... If a cabinet is made stiff enough, the cabinet resonances are above the frequency where music is likely to excite them. Furthermore, at the higher frequencies, the cabinet material has pretty effective internal damping.
I suspect that there is a resistance to accept the high-stiffness approach because it seems counter-intuitive.... The thinking goes something like this: Energy pumped into the cabinet walls by the driver is either radiated, or it is absorbed. Adding stiffness does not help absorb the energy... only by damping the vibrations and converting them into heat do we prevent re-radiation of that structural vibration as unwanted sound.
If speaker was required to produce continuous sine wave or noise signals, this argument would be (basically) true. However, that is not what speakers do.
The reason the high stiffness approach works is two-fold: (1) the vibration forcing function is music or movie soundtrack... which contains lots of transients. The frequency range of music that contains continuous high energy is approximately 50 Hz to 500 Hz. Above that range, the energy comes in short bursts. (2) second reason high stiffness works... At higher frequencies, all structures have increasing self damping. Plywood, MDF, and most other common materials have a lot more damping at 1 kHz than they do at 100 Hz. Same is true for glues and adhesives.
Planet10 has made this argument quite a few times in quite a few threads... If a cabinet is made stiff enough, the cabinet resonances are above the frequency where music is likely to excite them. Furthermore, at the higher frequencies, the cabinet material has pretty effective internal damping.
Even more thoughts:
The most fool-proof way to construct a non-resonant cabinet is the high-mass brute force method. This is what i did in my early days... multiple layers of cabinet wall and baffle materials, 1.5 inch thick walls, 3 inch thick baffles, which is very heavy. Concrete enclosures are an example of this also. If the cabinet walls have enough mass, there simply is no accoustic radiation from them.
This method works, but it is so heavy and unwieldy. For large cabinets (bigger than 100 liters), it is just not practical to have 200+ lb cabinets.
The most fool-proof way to construct a non-resonant cabinet is the high-mass brute force method. This is what i did in my early days... multiple layers of cabinet wall and baffle materials, 1.5 inch thick walls, 3 inch thick baffles, which is very heavy. Concrete enclosures are an example of this also. If the cabinet walls have enough mass, there simply is no accoustic radiation from them.
This method works, but it is so heavy and unwieldy. For large cabinets (bigger than 100 liters), it is just not practical to have 200+ lb cabinets.
I find this surprising. I had assumed that anyone with a formal engineering background in vibration (i.e. those having studied the effects of mass, damping and stiffness on vibrating structures) would know why a resonating stiff cabinet is generally no quieter than an equivalent resonating less stiff one.30 years as an aircraft structural engineer, dealing with both static loads and dynamic loads (i.e. vibration loads), leads me to believe in the high stiffness approach to cabinet construction.
At resonance the force due to mass and the force due stiffness are equal and opposite and cancel. It doesn't matter how stiff or heavy the cabinet is because the forces cancel leaving only the relatively weak damping force to limit the strength of resonances. If you have cabinet resonances present in the passband of the relevant driver/s then it is damping that largely determines the performance of the cabinet. The baffle is an exception where increasing stiffness will reduce the energy transferred into the cabinet but for the other panels it is damping that will remove energy from the vibrating cabinet (as will unwanted sound radiation of course) not stiffness or mass.
A subwoofer cabinet can be different if it is made stiff enough to raise the frequency of the lowest cabinet resonance well above the passband of the driver. So long as the subwoofer cabinet is then isolated from the woofer cabinet it's resonances won't get driven and there is no need for damping. This is a special case not the general case where the frequency of cabinet resonances will fall within the passband of the driver/s.
..At resonance the force due to mass and the force due stiffness are equal and opposite and cancel....
What seems like basic truthy Ph.D. physics is actually out-to-lunch when you check the details.
1. In the real world, there is a great difference between the freq band of resonance of a more-flimsy box versus a more-stiff box and also the degree of motion of each.
2. While I don't know the proportions, it seems the wall shaking we are concerned about comes from the stimulus of the pressure in the box rather than from panel resonance vibration and is only an issue for subs.
B.
A possible problem that comes with raising the stiffnes and thus the resonating frequency, is, our hearing is more sensitive in the 2-5 khz. So making a box resonate higher can be more audible than a lossy panel vibrating at a lower frequency. Assuming amplitude stays the same.
What seems like basic truthy Ph.D. physics is actually out-to-lunch when you check the details.
Understanding resonance is undergraduate level engineering not postgraduate engineering. The KEF white papers contain examples of how an engineer would go about engineering a speaker cabinet. Obviously they are primarily marketing exercises but the snippets of engineering are both genuine and representative of how the larger established speaker manufacturers design cabinets.
1. In the real world, there is a great difference between the freq band of resonance of a more-flimsy box versus a more-stiff box and also the degree of motion of each.
Not sure of your point. The lowest typical cabinet resonance varies from perhaps 100 Hz to 1 kHz depending on cabinet type and design approach. There are progressively more resonances above the lowest frequency.
Given a particular force a flimsy cabinet will deflect more than a stiff one but the lowest resonant frequency of a flimsy cabinet will be less than a stiff one. Since it requires less deflection at higher frequencies to create a given level of sound (e.g. the deflection of woofer and a tweeter for the same SPL) the SPL of the lowest resonance tends to remain about equally as loud. Here are some measurements showing that a stiffer cabinet created by doubling the cabinet wall thickness does not reduce the sound radiated by a cabinet.
2. While I don't know the proportions, it seems the wall shaking we are concerned about comes from the stimulus of the pressure in the box rather than from panel resonance vibration and is only an issue for subs.
Internal pressure is largely unimportant because it only becomes significant in size at low frequencies which are typically well below the frequency of cabinet resonances. At resonant frequencies it is small-to-negligible compared to the reaction forces from the drivers bolted to the baffle.
I find this surprising. I had assumed that anyone with a formal engineering background in vibration …..
I was surprised as well... when I got out of university in 1989, having taken "theory of vibrations", "theory of plates and shells", and "advanced mechanics of materials"... I was expecting a more theoretical approach to the design of aircraft for vibration loads. The strength design (i.e. stress analysis) was heavily FEM based (FEM = finite element model), and the distribution of static loads (aerodymic loads, inetia loads, etc) was based on FEM-derived stiffness... why would vibration loads be different? well, they were. Vibration loads analysis was almost entirely empirical. The very smart engineers in the dynamic loads department explained to me that the system complexity was just too high to design structure using models alone. Ground tests were used to determine the various resonant frequencies and modal shapes, and flight tests were used to determine the vibration environment that different areas of the aircraft would be subjected to. Dynamic modelling has come a long way, but things are still very empirical.
...It doesn't matter how stiff or heavy the cabinet is because the forces cancel leaving only the relatively weak damping force to limit the strength of resonances. If you have cabinet resonances present in the passband of the relevant driver/s then it is damping that largely determines the performance of the cabinet....
You speak as if this is all theoretical... as if we were all contemplating building a speaker cabinet for the first time and trying to decide how to do it. But it is not theoretical... many great examples of excellent cabinets have been built, going back over several decades. We know what works: Answer - all of the methods under discussion have produced excellent sounding cabinets. Massive brute force cabinets, highly damped cabinets, high stiffness cabinets, CLD cabinets. MDF cabinets, plywood cabinets, fiberglass, high density polymer, concrete, aluminum, concrete...
My point is that, while any of these methods can produce a great cabinet, the high stiffness approach is more likely to get it right the first time than a CLD or high damping approach. I think I speak for most DIY-ers in that I don't want to build multiple prototypes before I definitize the end product... no, I want to craft a cabinet, and be done. The high-stiffness approach gives me confidence I can get a good cabinet on the first try.
And as I said also... the most fool-proof way to build a great cabinet is to simply make it massive and well braced. Something like a double-wall filled with concrete, or 60 mm of MDF. If you can tolerate the weight, this is the KISS method. I can't do it however. I need to be able to move my speakers up and down stairs.
You speak as if this is all theoretical...
Basic engineering/scientific knowledge like what types of forces reduce a vibrating motion (e.g. damping) and what forces don't (e.g. mass and stiffness) may be theoretical but it is also true.
My point is that, while any of these methods can produce a great cabinet, the high stiffness approach is more likely to get it right the first time than a CLD or high damping approach.
This is not true. A stiff cabinet with little damping will resonate audibly. You may not recognise the sound but it will be present. To reduce the radiated sound below audible levels the loudest modes (i.e. the ones that are driven and radiate effectively to the listener) need to be reduced in level by effective damping.
Damping dissipates vibrating energy whereas stiffness and mass do not. A very heavy very stiff bell for example would be very noisy if you bolted some drivers to it. No doubt many DIY folk would know that it would be quiet because it is very stiff and very heavy but that won't change the reality that it would be noisy.
Why does it have to be one or the other? 🙂
Make it as stiff as you can and then use damping.
But considering how to implement both before is probably a good idea, some things can solve two problems at the same time or at least not make it worse.
The first speakers I built as a teenager was made from regular particleboard without bracing, and they were not That bad. Low frequency radiation that was acceptable.
But having built stiffer with MDF and MDF/Composite mix sure is better once bracing and bitumen pads are added.
When I worked with engine testing on an industrial level we encountered several problems with brackets that cracked of vibrations, thicker didn't help, it was a matter of reinforcing parts of it and removing material in other places. Diverting energy in to planes where there is strength and dampen the other places so it doesn't build too high amplitude.
Sometimes it helped just removing mass, but then again, not breaking and having good sound quality in your home could be two very different things.
Interesting subject at least. 🙂
Make it as stiff as you can and then use damping.
But considering how to implement both before is probably a good idea, some things can solve two problems at the same time or at least not make it worse.
The first speakers I built as a teenager was made from regular particleboard without bracing, and they were not That bad. Low frequency radiation that was acceptable.
But having built stiffer with MDF and MDF/Composite mix sure is better once bracing and bitumen pads are added.
When I worked with engine testing on an industrial level we encountered several problems with brackets that cracked of vibrations, thicker didn't help, it was a matter of reinforcing parts of it and removing material in other places. Diverting energy in to planes where there is strength and dampen the other places so it doesn't build too high amplitude.
Sometimes it helped just removing mass, but then again, not breaking and having good sound quality in your home could be two very different things.
Interesting subject at least. 🙂
Interesting discussion it is on the last pages, but I'm more a fan of the thin wall mass damped lossy cabinet principle that was pioneered by the BBC and is still used with the infamous Harbeth loudspeakers. I've build a few cabinets myself within this principle and those are the best speakers i've ever made. 12mm plywood for top, sides and bottom, 18mm for the front and back, and those are screwed on with rubber isolation strips between the front and the frame. The case is heavy braced with an 18mm plywood frame (strips of 30mm wide) and top, bottom and sides are damped with 4mm bitummen. This works very good for all but subwoofers as it brings the resonance frequency down in frequency (out the passband when done right) and amplitude. i'm totally turned to this way of working. Only for subs i still use full 18 or 21mm plywood, and MDF is not used anymore but for rough test-of-concept boxes. I actually also hate working with MDF and all the dust it creates. I'm no engineer, i'm an IT guy, so i do not have all the info why this works, but it does for me.
I bought a pair of home made corner cabs at a church sale and turned them into subs. There is no bracing just 2 layers of 3/4 inch plywood. Those are the deadest cans iv ever worked with. Put a glass of water on top and crank it up and the water is totally still.
For a given cabinet shape and dimension, it is a pretty straightforward process to design a bracing scheme that will result in a non-resonant cabinet.
HiFiJim’s comment on the extra complexity of a CLD cabinet is spot-on. It works. It is hard to do. And there is an easier way.
It is important to note that all speaker boxes resonant to some extent. The idea of this approach is to push any potential resonances up in frequency, and up in Q so that the energy needed to start the resonance has a very narrow bandwidth. This makes them almost impossible to get going when playing music.
Also mentioned was accelerometer measures, this is tedious, really best in an anechoic environment, and one has to do ab aweful lot of math & interpretation.
For thw diyer, a simpe mechanics stethescope is a cheap ($7-20), and easy to use tool that can quickly tell you where your panels are vibrating when playing music.
dave
I suspect that there is a resistance to accept the high-stiffness approach because it seems counter-intuitive...
It certainly breaks a lot of the “common” knowledge “rules” around speakers.
The journey to get where i am w speaker boxes started in the late ‘70s, when John Greenbank described his speaker box building philosophy and pointed out that if a reasonance is not excited it is as if it does not exist.
We have built literally 100s of boxes that use this approach and it turns out to work.
dave
At resonance the force due to mass and the force due stiffness are equal and opposite and cancel. It doesn't matter how stiff or heavy the cabinet is because the forces cancel leaving only the relatively weak damping force to limit the strength of resonances. If you have cabinet resonances present in the passband of the relevant driver/s then it is damping that largely determines the performance of the cabinet.
This is only true if there is sufficient energy at the resonance to excite the resonance, If that does not happen — ie, get the frequency (and Q) of any potential resonance so high that there is never (or rarely) enuff sustained hifrequency, near single note, energy to excite the (potential) resonance.
There is a resonance, but it is never excited, so it is as if it is not there.
dave
2. While I don't know the proportions, it seems the wall shaking we are concerned about comes from the stimulus of the pressure in the box rather than from panel resonance vibration and is only an issue for subs.
The pressure inside the box onlt transmits a small amount of energy into the box, and is only really an issue at the lowest frequencies where the internal damping/stuffing does not deal with it. Given that, less than half the driver’s energy is avaialble to be conveyed thru the pressure inside the box. It is easy to get stiffness up sufficiently to make this a non-issue (we have built 15mm sub boxes to test this).
Most of the energy loaded into the box is from the reactive forces acting on the basket, and by extension the baffle & the box.
With the big holes in it, the baflfe is typically the weakest panel (because of the holes we put in it to mount the drivers), This leads to the thick baffles we often see. I use a different approach, typically, tying the baffle, the driver itself, the top, bottom and back into a tightly coupled box section that distributes driver energy across 4 panels and the brace to reduce the instantaneous amount of energy pumped into any specific part of the box structure, and significantly increasing the rigidity of the whole box.
dave
The KEF white papers contain examples of how an engineer would go about engineering a speaker cabinet.
I have been sceptical of KEF’s box engineering since the fiasco of the flexy mounting of the drivers in the 1st KEF 105. An engineering solution that measures well and doesn’t work.
Not sure of your point. The lowest typical cabinet resonance varies from perhaps 100 Hz to 1 kHz depending on cabinet type and design approach.
And the amount of energy availble to excite the boxes drops with frequency. If you can move them up into the highest ov=ctave of that band you are getting into the area where there is insufficient energy to get things moving.
Something liike this (no calibration for the Y-axis):
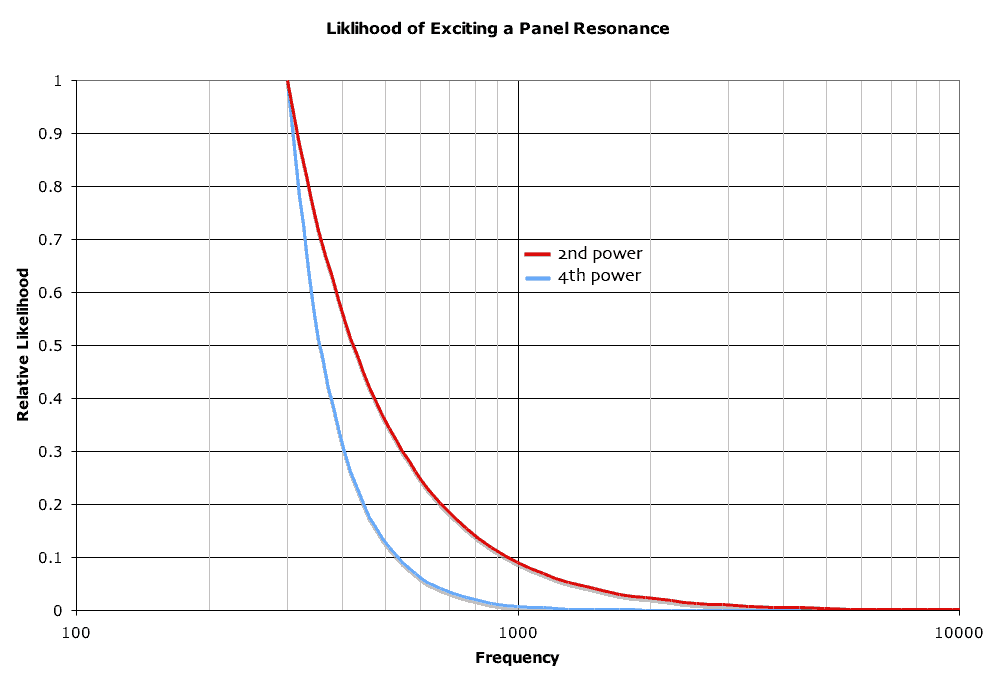
[/QUOTE]Internal pressure is largely unimportant because it only becomes significant in size at low frequencies which are typically well below the frequency of cabinet resonances. [/QUOTE]
:^)
dave
... why would vibration loads be different? well, they were. Vibration loads analysis was almost entirely empirical. The very smart engineers in the dynamic loads department explained to me that the system complexity was just too high to design structure using models alone.
ie, the vibration issue gets us into complexity theory, it is one of the 95% of real-world problems not solvable with the math available (before complexity theory — still in its infancy, but getting better (a good exampel is weather prediction)).
dave
A stiff cabinet with little damping will resonate audibly.
Only if the potential resonances are excited. I have many many boxes that would fit into this niche and. with music, they do not resonate.
dave
but I'm more a fan of the thin wall mass damped lossy cabinet principle that was pioneered by the BBC and is still used with the infamous Harbeth loudspeakers. I've build a few cabinets myself within this principle and those are the best speakers i've ever made. 12mm plywood for top, sides and bottom, 18mm for the front and back, and those are screwed on with rubber isolation strips between the front and the frame.
This has been proven to work pretty well. The BC1 is still a favorite of mine.
This technique pushes resonances down in frequency. Trying to move it below the voices.
But when i refurbed a friend’s Roger’s LS3/5A and compared them to a similar size mFonken, it was clear that the BBC approach did not work as well as my approach. In comparison the LS3/5a sounded boxy.
dave
- Home
- Loudspeakers
- Multi-Way
- Is MDF or plywood better for speaker cabinets?